REIDsteel
Structural Resilience
Steel buildings, designed, fabricated & built for
Maximum Hurricane Resilience
In the Caribbean, REIDsteel’s buildings have withstood thousands of hurricanes across hundreds of structures without a single known structural failure.
This remarkable track record speaks volumes about our commitment to resilience and quality.
Adapting to a Changing Climate
Over the past 50 years, climate change has dramatically altered weather patterns in the Caribbean, Southeast Asia, and the Indian Ocean. With wind speeds reaching unprecedented levels, many structures have failed to meet the challenge. However, REIDsteel’s buildings are designed to exceed Miami Dade wind speed recommendations, ensuring they remain standing even under extreme conditions.
Comprehensive Design for Ultimate Resilience
Our expertise goes beyond standard codes and practices. We meticulously consider every detail, from bracing techniques to corrosion protection. This ensures that our buildings can withstand both hurricanes and seismic activity.
Our structures are engineered to endure damage without compromising their integrity, even when faced with unexpected loads and impacts.
Proven Performance Over Decades
Our buildings, some over 50 years old, have survived the transition from Category 3 to Category 5 hurricanes, demonstrating their enduring strength. This longevity and resilience are a testament to our experience and dedication.
Experience That Sets Us Apart
There is no substitute for the experience we bring to hurricane-resistant construction. Our designs are not just about meeting codes—they are about exceeding expectations and ensuring safety and durability in the harshest of environments.
Tel: +44 (0) 1202 483333 • Email:
enquiries@reidsteel.co.uk
Tailored Solutions for Seismic Safety
Every region and every project presents unique challenges, especially when it comes to seismic activity. At REIDsteel, we understand that a one-size-fits-all approach does not work for seismic resilience. That’s why we offer tailored solutions to meet your specific needs. If your region has experienced structural damage due to seismic activity, let us provide you with a safe and reliable solution. Our team of experts will work closely with you to understand your requirements and design a structure that ensures the highest levels of safety and durability.
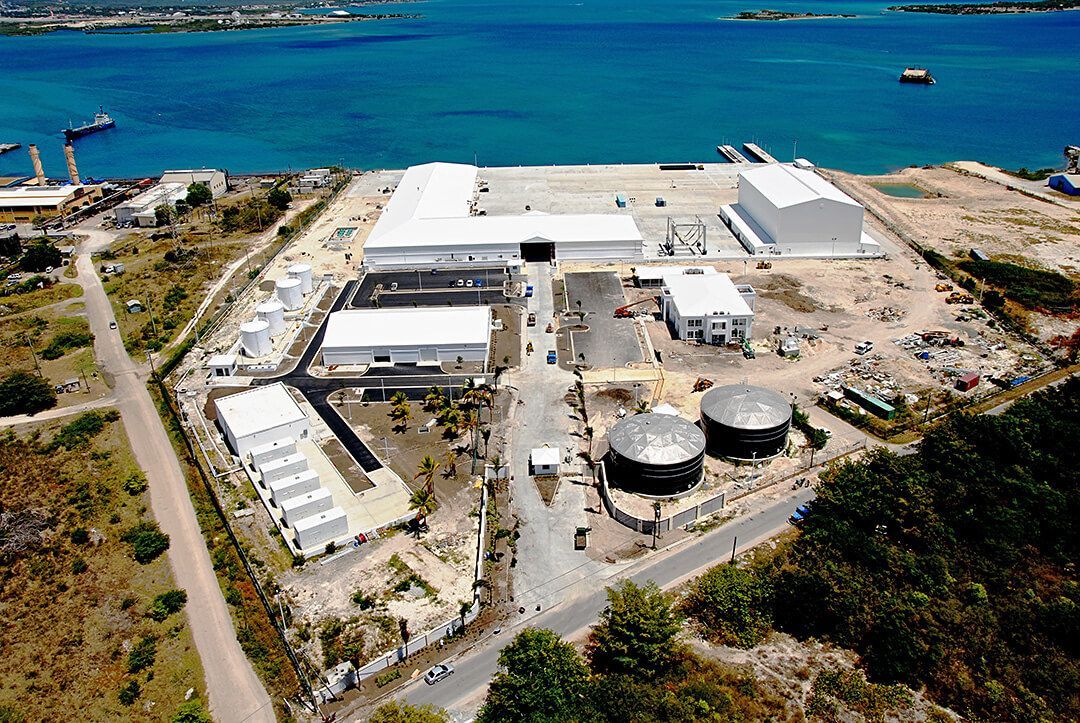
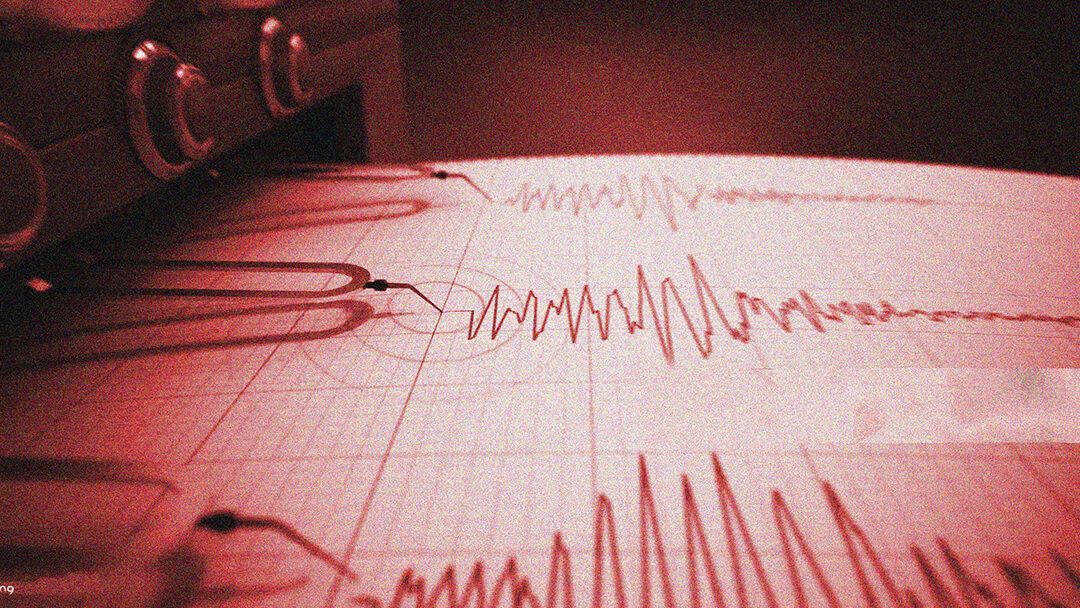
Tel: +44 (0) 1202 483333 • Email: enquiries@reidsteel.co.uk
Steel buildings, designed, fabricated & built for
Maximum Seismic Resilience
At REIDsteel, our commitment to seismic resilience is unparalleled.
We have constructed hundreds of buildings in some of the world’s most active seismic regions, and to our knowledge, none have suffered a structural failure.
This impeccable record is a testament to our expertise and dedication to creating buildings that can withstand the most severe seismic forces. Our approach ensures that every structure we design is not only robust but also capable of protecting lives and assets during an earthquake.
Innovative Engineering Solutions
The challenge of modern structural engineering lies in balancing cost, efficiency, and resilience. Many modern analysis packages and codes struggle with plastic design, a method that offers significant cost and weight savings for ordinary structures. However, the Structural Eurocodes have been written to limit the use of plastic design, which is crucial for efficient seismic resilience. At REIDsteel, our expert Structural Engineers have mastered the art of combining traditional knowledge with the latest scientific advancements. By integrating the principles of both BS EN1998 (Eurocode) and ASCE 7-22 (American) standards, we ensure that our buildings are designed to the highest standards of seismic resilience.
Proven Performance Across the Globe
Our stellar track record is not confined to a single region or type of structure. We have successfully designed and constructed a wide range of buildings across many countries, each tailored to meet the specific seismic challenges of its location. Our buildings often exceed the stringent requirements for seismic resilience, providing peace of mind to our clients. Whether it’s a commercial building, a residential complex, or a critical infrastructure project, our designs are crafted to endure the forces of nature and protect what matters most.
Steel buildings, designed, fabricated & built for
Maximum Blast Resilience
At REIDsteel, we have spent decades perfecting our blast mitigation systems in collaboration with armed forces around the globe. Our extensive experience has led to the development of advanced stand-off systems that effectively protect against both accidental explosions and deliberate attacks. One of our standout innovations is our low-weight, low-cost mortar roof design, which has been widely implemented in Afghanistan. This design not only demonstrates our commitment to safety and efficiency but also highlights our ability to adapt to the unique challenges of different environments.
Adapting to Modern Threats
In today’s rapidly evolving threat landscape, the techniques we developed for anti-mortar structures have proven to be highly effective against new dangers, such as drone-borne explosives. Our blast mitigation strategy is built on four key principles: extending the distance to the blast point, minimising the creation of additional shrapnel, resisting the blast wave at an appropriate distance, and preventing spalling behind that point. These principles ensure that our mortar defence roofs are not only effective against traditional threats but also provide robust protection against modern, low-speed drone attacks.
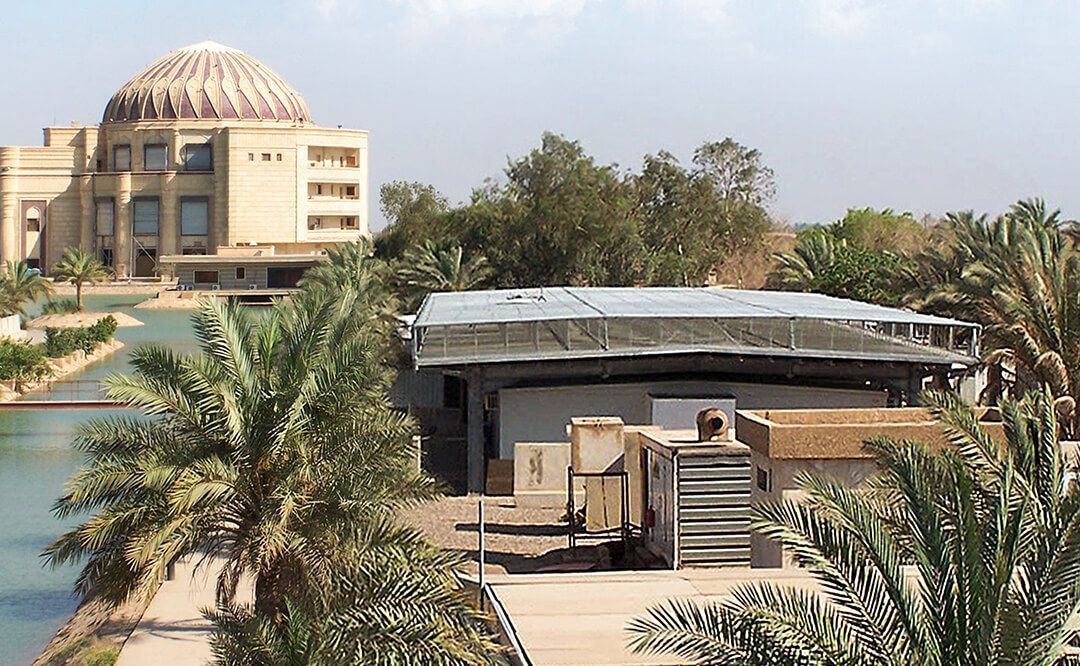
Tailored Solutions for Your Safety
We understand that every threat and asset is unique. That’s why we offer tailored solutions to meet your specific needs. Whether you are concerned about protecting a particular asset or facing a specific threat, our team of experts is ready to assist you. We leverage our extensive experience and cutting-edge technology to design blast-resistant structures that ensure the highest levels of safety and durability.
Tel: +44 (0) 1202 483333 • Email:
enquiries@reidsteel.co.uk
Innovative Dragon’s Teeth Design
Originally designed for military applications, our Dragon’s Teeth system was created to be easy to deploy without foundations, simple to assemble, cost-effective, and highly resistant to high-speed vehicle attacks. To enhance their versatility, we designed a range of gates that fit seamlessly within the cast concrete retainers (teeth).
Rigorous Testing and Proven Performance
We subjected the Dragon’s Teeth and gates to extensive vehicular (7.5t truck) and blast testing. The results confirmed that our design meets the highest standards of perimeter protection, making it ideal for commercial operations with vulnerable boundaries, such as airports and the energy and utility sectors.
Hostile vehicle mitagation gates • designed & fabricated for
Maximum HVM Resilience
Through extensive testing to BSi PAS 68 standards, REIDsteel has developed a robust system of gates (both mobile and founded) and moveable barriers that can withstand impacts from both high and low-speed threats, including fully laden HGVs.
Our expertise was notably demonstrated in the defense of all venues for the London 2012 Olympic Games.
Ideal for Temporary and Reusable Protection
The Dragon’s Teeth & Gates have also become popular with major event organisers. Their foundation-free design makes them perfect for temporary and reusable perimeter protection solutions. Additionally, we offer customizable covers for the Dragon’s Teeth that can be branded for sponsorship or promotional purposes.
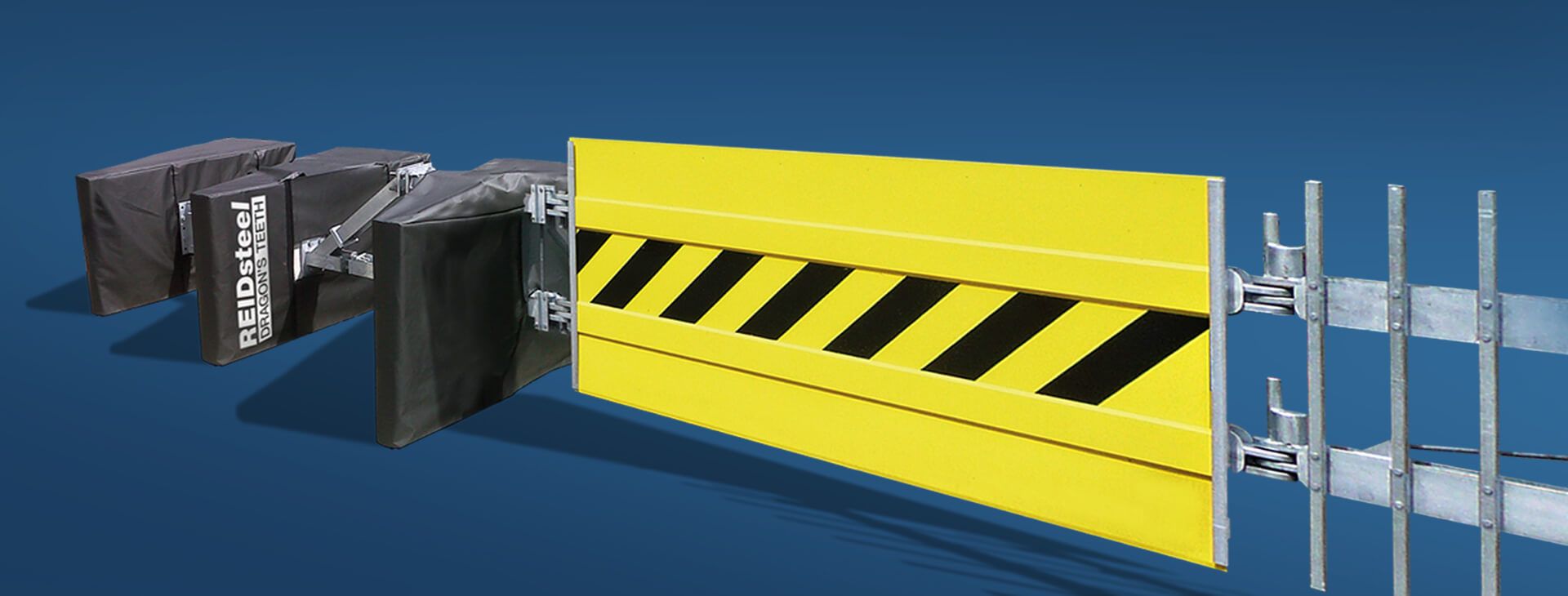
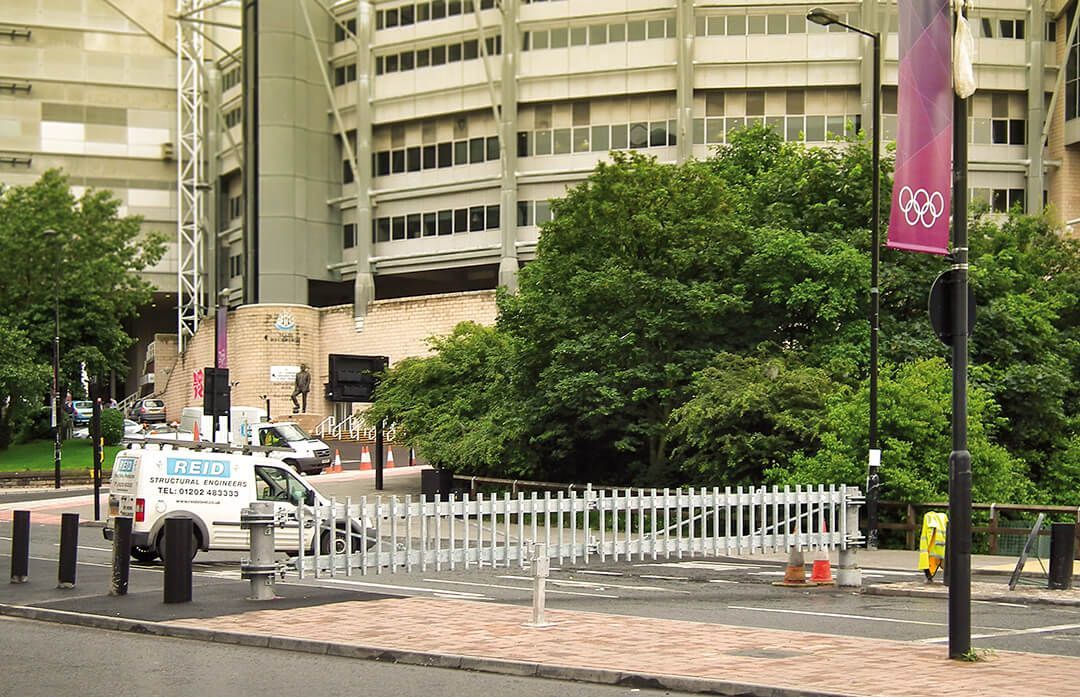
Choose REIDsteel for high-security vehicle mitigation solutions that combine innovation, reliability, and versatility.
Tel: +44 (0) 1202 483333 • Email:
enquiries@reidsteel.co.uk
Plus hurricane & seismic resilient buildings, stadia & grandstands, HVM gates, hospitals, housing, defence structures, car parks . . .